Return to MODULE PAGE
Neurons, Synapses, Action Potentials, and Neurotransmission
Robert Stufflebeam: Author, Artist & Animator
Functions of Neurons
The central nervous system [CNS] is composed entirely of two kinds of specialized cells: neurons and glia. Hence, every information processing system in the CNS is composed of neurons and glia; so too are the networks that compose the systems (and the maps). Clearly, without these two types of cells, the CNS would not be able to do what it does (which is everything having to do with our minds and how we move our bodies). But what do neurons and glia themselves do? What are their functions?
Neurons are the basic information processing structures in the CNS. Everything occurring above the level of neurons qualifies as information processing too. But nothing below the level of neurons does. We shall ignore that this view, called the neuron doctrine, is somewhat controversial. What isn't controversial is that the function of a neuron is to receive INPUT "information" from other neurons, to process that information, then to send "information" as OUTPUT to other neurons. (Synapses are connections between neurons through which "information" flows from one neuron to another.) Hence, neurons process all of the "information" that flows within, to, or out of the CNS. All of it! All of the motor information through which we are able to move; all of the sensory information through which we are able to see, to hear, to smell, to taste, and to touch; and of course all of the cognitive information through which we are able to reason, to think, to dream, to plan, to remember, and to do everything else that we do with our minds. Processing so many kinds of information requires many types of neurons; there may be as many as 10,000 types of them. Processing so much information requires a lot of neurons. How many? Well, "best estimates" indicate that there are around 200 billion neurons in the brain alone! And as each of these neurons is connected to between 5,000 and 200,000 other neurons, the number of ways that information flows among neurons in the brain is so large, it is greater than the number stars in the entire universe!
While we are considering numbers, it is worth noting that there are as many as 50 times more glia than neurons in our CNS! Glia (or glial cells) are the cells that provide support to the neurons. In much the same way that the foundation, framework, walls, and roof of a house prove the structure through which run various electric, cable, and telephone lines, along with various pipes for water and waste, not only do glia provide the structural framework that allows networks of neurons to remain connected, they also attend to the brain's various house keeping functions (such as removing debris after neuronal death).
Because our main interest lies in exploring how information processing occurs in the brain, we are going to ignore glia. But before we see how neurons process information (and what that means), you need to know a few things about the structure of neurons.
Structure of neurons
While there are as many as 10,000 specific types of neurons in the human brain, generally speaking, there are three kinds of neurons: motor neurons (for conveying motor information), sensory neurons (for conveying sensory information), and interneurons (which convey information between different types of neurons). The following image identifies how neurons come in various shapes and sizes. (It is based on drawings made by Cajal.)
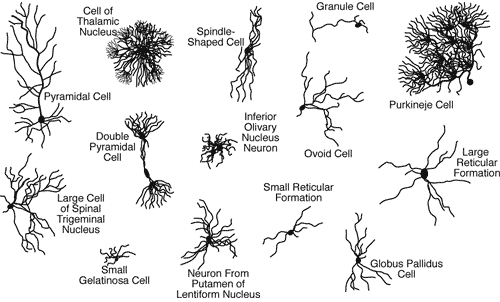
A "typical" neuron has four distinct parts (or regions). The first part is the cell body (or soma). This is not only the metabolic "control center" of the neuron, it is also its "manufacturing and recycling plant." (For instance, it is within the cell body that neuronal proteins are synthesized.) The second and third parts are processes — structures that extend away from the cell body. Generally speaking, the function of a process is to be a conduit through which signals flow to or away from the cell body. Incoming signals from other neurons are (typically) received through its dendrites. The outgoing signal to other neurons flows along its axon. A neuron may have many thousands of dendrites, but it will have only one axon. The fourth distinct part of a neuron lies at the end of the axon, the axon terminals. These are the structures that contain neurotransmitters. Neurotransmitters are the chemical medium through which signals flow from one neuron to the next at chemical synapses.
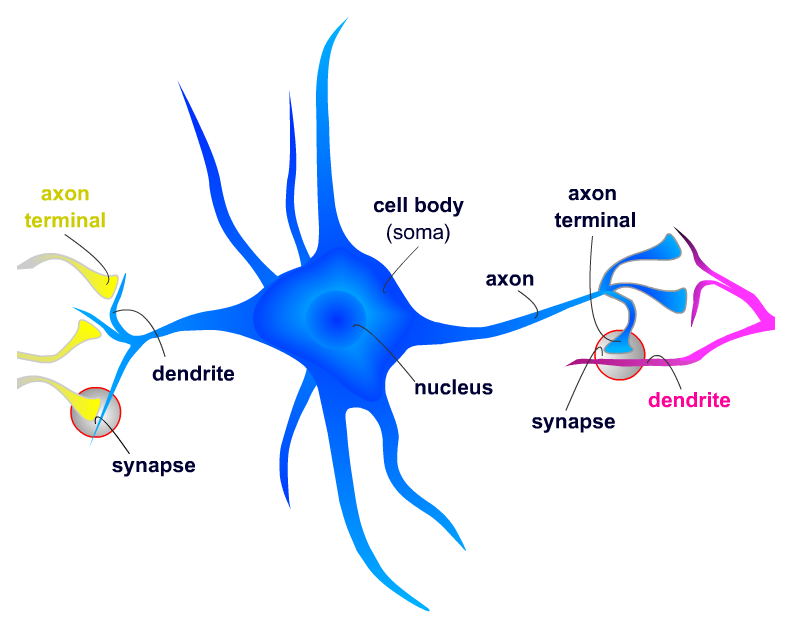
Neuronal signaling
To support the general function of the nervous system, neurons have evolved unique capabilities for intracellular signaling (communication within the cell) and intercellular signaling (communication between cells). To achieve long distance, rapid communication, neurons have evolved special abilities for sending electrical signals (action potentials) along axons. This mechanism, called conduction, is how the cell body of a neuron communicates with its own terminals via the axon. Communication between neurons is achieved at synapses by the process of neurotransmission.
Conduction
To begin conduction, an action potential is generated near the cell body portion of the axon. An action potential is an electrical signal very much like the electrical signals in electronic devices. But whereas an electrical signal in an electronic device occurs because electrons move along a wire, an electrical signal in a neuron occurs because ions move across the neuronal membrane. Ions are electrically charged particles. The protein membrane of a neuron acts as a barrier to ions. Ions move across the membrane through ion channels that open and close due to the presence of neurotransmitter. When the concentration of ions on the inside of the neuron changes, the electrical property of the membrane itself changes. Normally, the membrane potential of a neuron rests as -70 millivolts (and the membrane is said to be polarized). The influx and outflux of ions (through ion channels during neurotransmission) will make the inside of the target neuron more positive (hence, de-polarized). When this depolarization reaches a point of no return called a threshold, a large electrical signal is generated. This is the action potential. How it is generated is illustrated in the following animation.
THE ACTION POTENTIAL
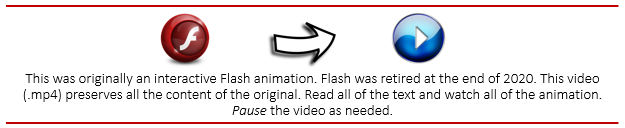
This signal is then propagated along the axon (and
not, say, back to its dendrites) until it reaches its axon terminals. An
action potential travels along the axon quickly, moving at rates
up to 150 meters (or roughly 500 feet) per second. Conduction
ends at the axon terminals. Axon terminals are where neurotransmission begins.
Neurotransmission
Neurotransmission (or synaptic transmission) is communication between neurons as accomplished by the movement of chemicals or electrical signals across a synapse. For any interneuron, its function is to receive INPUT "information" from other neurons through synapses, to process that information, then to send "information" as OUTPUT to other neurons through synapses. Consequently, an interneuron cannot fulfill its function if it is not connected to other neurons in a network. A network of neurons (or neural network) is merely a group of neurons through which information flows from one neuron to another. The image below represents a neural network. "Information" flows between the blue neurons through electrical synapses. "Information" flows from yellow neuron A, through blue neuron B, to pink neuron C via chemical synapses.
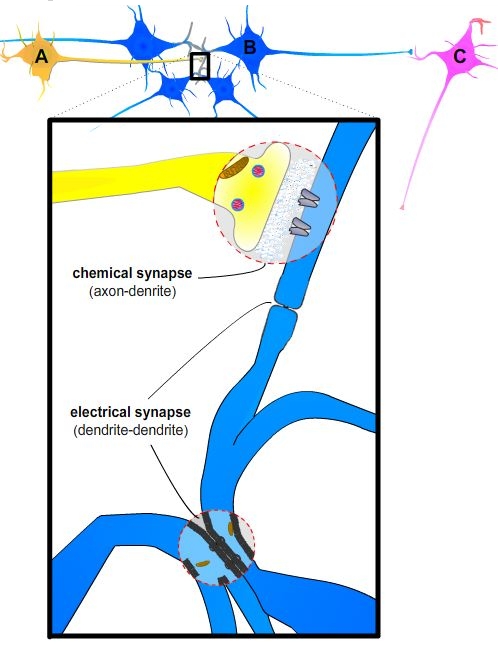
The following animation illustrates the difference between these two kinds of synapses.
SYNAPSES
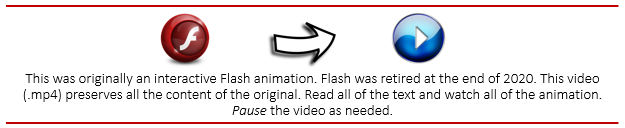
At electrical
synapses,
two neurons are physically connected to one another through gap junctions.
Gap junctions permit changes in the electrical properties of one neuron to
effect the other, and vice versa, so the two neurons essentially behave as
one.
ELECTRICAL NEUROTRANSMISSION
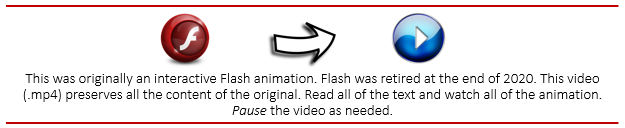
Chemical
neurotransmission occurs at chemical synapses. In chemical
neurotransmission, the presynaptic neuron and the postsynaptic neuron are
separated by a small gap — the synaptic
cleft. The synaptic cleft is filled with extracellular
fluid (the fluid bathing all the cells in the brain). Although very small,
typically on the order of a few nanometers (a billionth of a meter), the
synaptic cleft creates a physical barrier for the electrical signal carried
by one neuron to be transferred to another neuron. In electrical terms,
the synaptic cleft would be considered a “short” in an electrical
circuit. The function of neurotransmitter is
to overcome this electrical short. It
does so by acting like a chemical messenger, thereby linking the action
potential of one neuron with a synaptic potential in another. How this
occurs is illustrated in the following animation.
CLASSIC CHEMICAL NEUROTRANSMISSION
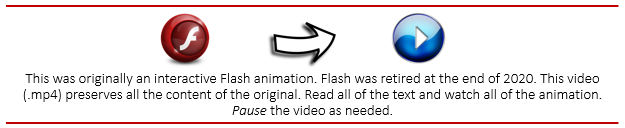