Virtual Cocaine Lab
BOOK 1: Nervous System Anatomy & Function
Paul Garris: Primary Author
Ch. 1: Nervous system anatomy
The nervous system performs many
tasks: it coordinates the activity of the muscles, it
monitors the organs, it processes input from the senses, and it initiates action. The brain and spinal cord comprise the central
nervous system. The central nervous system
is protected by bone (skull and vertebrae). The
peripheral nervous system is not because its function is to relay information
to and from the organs and the limbs. The peripheral nervous system consists of all the other structures that do not
lie within the central nervous system. These other structures include motor
neurons, which stimulate muscle tissue, and sensory neurons, which include
those connected to pain- or temperature-sensitive receptors in the skin.
Anatomically,
the brain is divided into three parts: forebrain, midbrain, and hindbrain (see Figure 1). These subdivisions control different functions. Not unexpectedly,
these parts come in varying sizes in different animals, depending upon specific
needs or features of the animal.
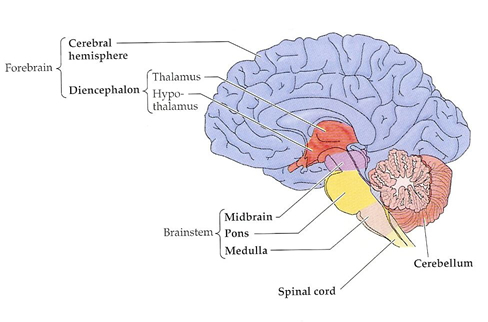
Fig. 1: Major divisions of the brain.
Collectively,
the cerebellum, medulla, and pons are called the hindbrain and perform "lower-level functions." The cerebellum
is the ball-like structure resting on the back of the brain. It controls fine
motor movement, coordination, and posture. Closest to the spinal cord, the
medulla controls breathing and heart beat. Above the medulla is the pons (or
"bridge"). It relays sensory information between the cerebellum and the
cerebrum. The midbrain (which
lies between the hindbrain and the forebrain) is involved in movement and
audio-visual processing. The medulla, pons, and midbrain comprise the brainstem.
The forebrain consists of the diencephalon and the two cerebral hemispheres
(or the cerebrum). The diencephalon
("in between" brain) is comprised of two prominent structures: the hypothalamus
and thalamus. The hypothalamus is located at the very bottom of the brain,
directly on top of the roof of the mouth. It controls a variety of involuntary
functions, including: blood pressure, temperature regulation, feeding, sexual
behavior, and the pituitary gland – the body's master gland. The thalamus
resides on top of the hypothalamus and is the major relay system in the brain.
All sensory information except smell first comes to the thalamus before being
sent to other regions for processing.
In humans, the
two cerebral hemispheres (or cerebrum) are by far the largest structure of the brain.
They are involved in cognition, memory, language processing, motor processing,
and sensory information processing. They also contain the basal
ganglia — the group of nuclei that
is central to the control of movement. Parkinson's disease and Huntington's
disease are diseases that result from damage to the basal ganglia.
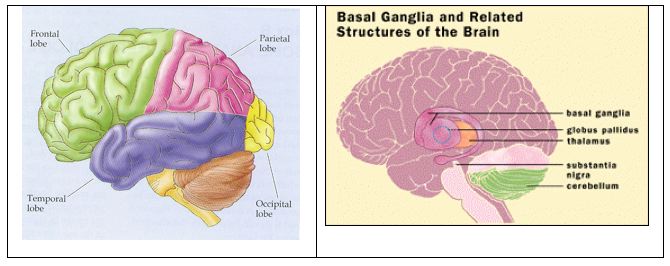
Fig. 2: Four lobes and basal ganglia.
Each cerebral hemisphere is
composed of four lobes: frontal, parietal, temporal, and occipital (see Figure
2). The frontal lobes are considered the site of executive decisions,
planning, and personality. They are also the primary seat of motor information
processing. The parietal lobes
are involved with attention and somatosensory (i.e., "body senses") information
processing. The temporal lobes
are involved in recognition and auditory information processing. The occipital
lobes, which have no cognitive function,
contain the primary visual information processing centers.
Ch. 2: Neuronal signaling
Neurons are the
basic information processing structures in the brain. A "typical"
neuron has four distinct parts (or regions). The first part is the cell body (or soma). This is not only the metabolic
"control center" of the neuron, it is also its "manufacturing
and recycling plant." (For instance, it is within the cell body that
neuronal proteins are synthesized.) The second and third parts are processes — structures that extend away from the cell
body. Generally speaking, the function of a process is to be a conduit through
which signals flow to or away from the cell body. Incoming signals from other
neurons are (typically) received through its dendrites. The outgoing signal to other neurons flows along
its axon. A neuron may have many
thousands of dendrites, but it will have only one axon. The fourth distinct
part of a neuron lies at the end of the axon, the axon terminals. These are the structures that contain
neurotransmitters. Neurotransmitters are the chemical medium through which signals flow from one neuron to
the next at chemical synapses.
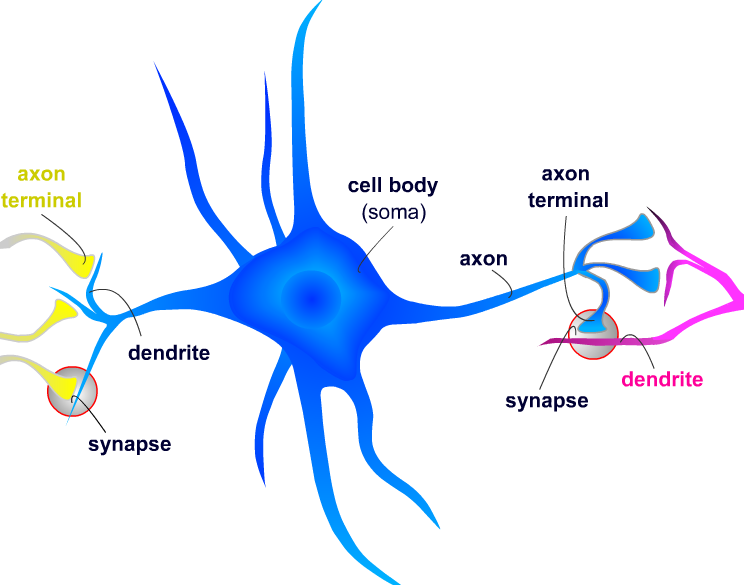
Fig. 3: Structure of a neuron.
To support the
general function of the nervous system, neurons have evolved unique
capabilities for intra-cellular signaling (communication
within the cell) and inter-cellular signaling (communication between cells). To achieve long
distance, rapid communication, neurons have evolved special abilities for
sending electrical signals (action potentials) along axons. This mechanism, called conduction, is how the cell body of a neuron communicates with
its own terminals via the axon. Communication between neurons is achieved at synapses
by the process of neurotransmission.
To begin conduction, an action potential is
generated near the cell body portion of the axon. An action potential is an
electrical signal very much like the electrical signals in electronic devices.
But whereas an electrical signal in an electronic device occurs because
electrons move along a wire, an electrical signal in a neuron occurs because ions move across the neuronal membrane.
GENERATION OF THE NEURAL IMPULSE
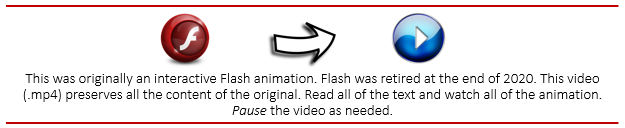
An
action potential propagates along the axon quickly, moving at rates up
to 150 meters (or roughly 500 feet) per second.
Conduction ends at the axon terminals. Axon terminals are where
neurotransmission begins.
Neurotransmission is communication of information between neurons as
accomplished by the movement of chemicals or electrical signals across a
synapse. There are two kinds of synapses (see Figure 4).
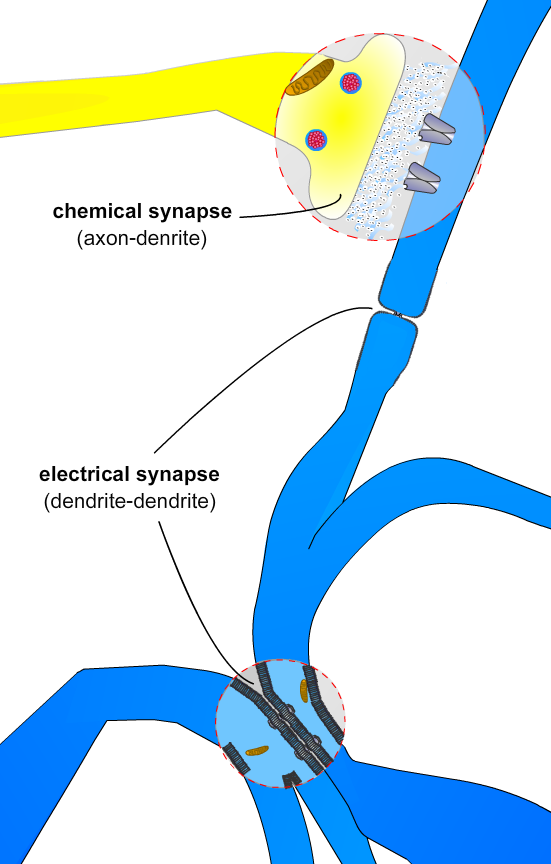
Fig. 4: Two types
of synapses.
At electrical synapses, two neurons are physically connected to one another through gap
junctions. Gap junctions permit changes in the electrical properties of one
neuron to effect the other, and vice versa, so the two neurons essentially
behave as one.
At chemical synapses,
two neurons are not physically connected to one another. As such, the arrival
of an action potential in the presynaptic neuron triggers the release of
chemical neurotransmitters. It is through chemical neurotransmitters that the
presynaptic neuron communicates with the postsynaptic neuron. In mammals, most
neurons communicate through chemical means. There are two models for how this
occurs; namely, "classic" chemical neurotransmission and volume neurotransmission. Here only the first will be discussed.
In "classic" chemical neurotransmission, the presynaptic neuron and the postsynaptic neuron are
separated by a small gap — the synaptic cleft. The synaptic cleft is filled with extracellular
fluid (the fluid bathing all the cells in the brain). Although very small,
typically on the order of a few nanometers (a billionth of a meter), the
synaptic cleft creates a physical barrier for the electrical signal carried by
one neuron to be transferred to another neuron. In electrical terms, the
synaptic cleft would be considered a "short" in an electrical circuit. The
function of neurotransmitter is to overcome this electrical short
.
CLASSIC CHEMICAL NEUROTRANSMISSION
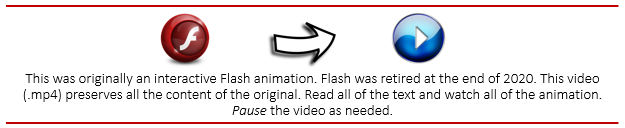
The entry of ions through ion channels produces a local partial depolarization (change in voltage) of the membrane. The postsynaptic neuron can have thousands of chemical synapses producing local partial depolarizations of its membrane (see Figure 5 ).
FIG. 5
When
the sum of all its partial depolarizations exceeds the threshold, the
postsynaptic neuron will fire an action potential, thus completing neuronal
signaling. It then becomes the presynaptic neuron to all the neurons it is
connected to via synapses at its axon terminals (see Conduction above).
Chemical
neurotransmission is terminated by removal of neurotransmitter from the cleft.
Besides through diffusion, this can occur in different ways. Some types of neurotransmitter
are removed by
degradative enzymes found in the
synaptic cleft. The function of these enzymes is to break down or deactivate
a neurotransmitter so that it can no longer bind to a receptor. However, for
most types of neurotransmitter, removal from the cleft is accomplished through
a special protein on the presynaptic neuron called a transporter. A transporter protein acts as a pump. It binds
neurotransmitter in a way that is similar to the way a receptor does, but then
it moves the neurotransmitter back into the neuron, a process called reuptake. Thus, for most neurons, the same neuron that
initiates neurotransmission by releasing neurotransmitter also terminates
neurotransmission by removing neurotransmitter. Once inside the neuron,
neurotransmitter molecules are either re-packaged into vesicles for use again
or deactivated and broken down via degradative enzymes found in the cell.
Ch. 3: Rats vs. Humans
Rats are often used in research
because of the similarities they have with humans. In rats, as in humans, the
nervous system is divided into the central nervous system and peripheral
nervous system. Rats and humans share all of the major subdivisions of the
brain and their general functions. And rats and humans also share similar
dopamine neuron systems. Dopamine is an
important neurotransmitter involved in movement and motivated behavior (see Book
2).
However, important differences exist between rats and humans. For example, humans have a considerably larger cerebral cortex, while rats have a more prominent olfactory bulb. This latter region of the forebrain is important for smell, a sense that is extremely keen in rats, but less so in humans. Another important difference is the physical relationship between the brain and the spinal cord. As an animal that stands upright, the brain and spinal cord in a human form a right angle, with the spinal cord extending down from the base of the brain. Because rats "stand" on all four paws, the relationship is more linear.