PARKINSON'S DISEASE: A Debate about its Nature & Cause
OVERVIEW OF THE MAIN CONTENT OF
The Mind Project's Virtual Neuroscience Lab #2: Parkinson's Study
CONTRIBUTORS:
Paul Garris: Project Leader, Author, Storyboards Kevin Stewart: Animations, Artwork, Programming, Audio/Video Engineering, Design David Leech Anderson: Author, Storyboards Robert Stufflebeam: Artwork, Author, Design |
Darci J. Harland: Storyboards Elisa L. Palmer: Author, Storyboards Andrew Kenning: Author, Storyboards Ji Zheng: Animations, Artwork |
MODULE COMPONENTS
PARKINSON'S DISEASE: A Debate about its Nature & Cause
NOTE #1: This html document covers much (but far from all) of the content of this virtual Parkinson's lab. If you have the time, we encourage you to immerse yourself in the complete lab. For teachers who want to use the virtual lab or the materials found in this document, you will find a number of helpful supporting materials at the Virtual Parkinson's Lab.
CONTENTS
- What is Parkinson's Disease?
- Symptoms of Parkinson's Disease
- Causes of Parkinson's Disease
- How Dopamine Neurotransmission Works
- Dopamine Tone & PD
- The Increased Release Theory
- Experiments to Test Increased Release Theory
- The Passive Stabilization Theory
- The Bucket Theory
- Computer Model of Passive Stabilization
- Three-Way Animation: Neurons, Computer Model & Bucket
1. What is Parkinson's Disease?
Parkinson's disease is a progressive neurological disorder that results from degeneration of neurons that produce dopamine in a region of the brain that controls movement. This degeneration creates a shortage of the brain
signaling chemical (neurotransmitter) known as dopamine, causing the movement impairments that characterize the disease. While Parkinson's Disease has been described since ancient times, it was first discussed in detail in a
medical journal in 1817 by Dr. James Parkinson. The disease was named after him some 60 years later by Dr. Jean Martin Charcot. While the symptoms of the disease have been documented for some time, the cause of Parkinson's
Disease is in part still a mystery.
Often, the first symptom of Parkinson's disease is tremor (trembling or shaking) of a limb, especially when the body is at rest. The tremor often begins on one side of the body, frequently in one hand. Other common symptoms
include slow movement (bradykinesia), an inability to move (akinesia), rigid limbs, a shuffling gait, and a stooped posture. People with Parkinson's disease often show reduced facial expressions and speak in a soft voice.
Occasionally, the disease also causes depression, personality changes, dementia, sleep disturbances, speech impairments, or sexual difficulties. The severity of Parkinson's symptoms tends to worsen over time.
In the United States, at least 500,000 people are believed to suffer from Parkinson's disease, and about 50,000 new cases are reported annually. These figures are expected to increase as the average age of the population
increases. The disorder appears to be slightly more common in men than women. The average age of onset is about 60. Both prevalence and incidence increase with advancing age; the rates are very low in people under 40 and rise
among people in their 70s and 80s. Parkinson's disease is found all over the world. The rates vary from country to country, but it is not clear whether this reflects true ethnic and/or geographic differences or discrepancies in
data collection.
Treatment of Parkinson's Disease varies from patient to patient but there are several drugs that seem to help with the symptoms of the disease. The most widely used one, Levadopa (L-dopa), is converted into dopamine by the body,
thereby increasing the amount of dopamine in the brain and decreasing the symptoms of the disorder. While there currently is no cure for Parkinson's disease, progress in the field is being made. One such promising treatment is
deep brain stimulation. In this procedure, an electrode is placed in the patient's brain and then stimulated by a battery pack. The electrical impulses seem to decrease or stop the physical symptoms of the disorder in most
patients.
A. Who Gets Parkinson's Disease?
- The elderly primarily get Parkinson's Disease
- 1 Million sufferers in the US
- 2% lifetime risk
- Some young adults get Parkinson's Disease
B. How is the Brain Damaged in Parkinson's Disease?
The brain loses the neurotransmitter Dopamine in the Substantia Nigra and Striatum.
C. What Causes Parkinson's Disease?
- The cause of most cases of Parkinson's Disease is unknown
- Genes and chemicals have been implicated in some cases
D. Can Parkinson's Disease Be Cured?
- No, but it can be treated with drugs and surgery
- Drugs that increase brain dopamine (levadopa) or mimic dopamine (agonists) are most commonly used
- The neurosurgical technique of Deep Brain Stimulation (DBS) is also being used more commonly
2. Symptoms of Parkinson's Disease
Parkinson's Disease is usually diagnosed after the appearance of one or more of its four most common motor symptoms: resting tremor, bradykinesia, rigidity, and postural instability. Parkinson's patients will exhibit any of
these symptoms in a wide range of severity. There are many more symptoms associated with Parkinson's Disease. These symptoms fall into three main categories: primary motor symptoms, secondary motor symptoms, and nonmotor symptoms.
Primary Motor Symptoms
- Resting Tremor - A "resting tremor" is called such because it appears as a slight tremor in a body part that is not at work and disappears when that body part begins an action. About 70% of all Parkinson's patients experience a resting tremor in the beginning stages of the disease.
- Bradykinesia - Bradykinesia refers to the slowing of a person's movements. Parkinson's patients can experience both slow movements and incomplete movements along with difficulty initiating movements or sudden halts to movements in progress. Bradykinesia in Parkinson's patients is often characterized by slow, shuffling steps and a reduction in facial expressions.
- Rigidity - Rigidity (increased muscle tone) leads to stiffness and inflexibility in the muscles. Muscles normally relax when they are at rest and, in the case of rigidity, the muscles never relax, leading to decreased range of motion as well as pain and cramping in some situations. The combination of bradykinesia and rigidity in facial muscles can lead to a "mask-like' look, due to a reduction in facial expressions.
- Postural Instability - Parkinson's patients often experience instability while tanding as well as reduced balance and coordination. This, in combination with bradykinesia, greatly increases the risk of falling and causes difficulty with making turns or sudden movements. These individuals may also experience "freezing", where they can not initiate walking and feel stuck.
Secondary Motor Symptoms
Secondary motor symptoms are called such because, while they are commonly found in cases of Parkinson's Disease, they are found in differing combinations and degrees of severity.
- Dystonia - Involuntary contractions of the muscles, leading to twisting and repetitive movements
- Fatigue
- Impaired Fine Motor Dexterity and Motor Coordination
- Impaired Gross Motor Coordination
- Poverty of Movement - Decreased arm swing
- Akathisia - Feelings of inner restlessness, resulting in the inability to stay still
- Speech Problems - Softness of voice or slurred speech caused by lack of muscle control
- Loss of Facial Expression, or 'Masking'
- Micrographia - Small, cramped handwriting
- Difficulty Swallowing
- Sexual Dysfunction
- Drooling
- Drooped Posture w/ Tendency to Lean Forward
Nonmotor Symptoms
In addition to the many motor symptoms commonly associated with Parkinson's Disease, there are many symptoms exhibited by Parkinson's patients that have nothing to do with movement, hence the term "nonmotor". Again, like the
motor symptoms, these nonmotor symptoms will be exhibited in different combination and in differing degrees of severity by different patients.
- Pain
- Dementia or Confusion
- Sleep Disturbances
- Constipation
- Skin Problems
- Depression
- Fear or Anxiety
- Memory Difficulties and Slowed Thinking
- Urinary Problems
- Fatigue and Aching
- Loss of Energy
- Compulsive Behavior
- Cramping
3. Causes of Parkinson's Disease
It was in the 1960s when the first chemical brain differences were detected in Parkinson's patients. It was discovered that patients showing symptoms of Parkinson's disease had low levels of dopamine in the striatum due to the
loss of dopamine producing neurons in the substantia nigra. The dopaminergic neurons in the substantia nigra have axons that project through the medial forebrain bundle and end in the striatum, where they release dopamine. This
pathway is known as the nigrostriatal tract. The release of dopamine in the striatum then stimulates a series of transmissions, beginning with the thalamus and ending with the motor cortex.
Further research determined that the Parkinson's patients had usually lost over 80% of their dopamine-producing neurons in the substantia nigra before they began showing visible symptoms. What was not known is why these dopamine
neurons begin to die or how the brain can maintain such a great loss of dopamine-producing neurons before showing visible symptoms.
There are several theories as to what causes the initial loss of dopamine-producing neurons in the substantia nigra and it is thought that the cause may vary between patients. It is thought that the disease may at times be the
result of genetics, chemicals, environmental toxins or any combination of the three. Individuals who develop symptoms before the age of 50 are thought to have a stronger genetic tendency than those who develop symptoms later in
life.
4. How Dopamine Neurotransmission Works
Often, the first symptom of Parkinson's disease is tremor (trembling or shaking) of a limb, especially when the body is at rest. The tremor often begins on one side of the body, frequently in one hand. Other common symptoms include slow movement (bradykinesia), an inability to move (akinesia), rigid limbs, a shuffling gait, and a stooped posture. People with Parkinson's disease often show reduced facial expressions and speak in a soft voice. Occasionally, the disease also causes depression, personality changes, dementia, sleep disturbances, speech impairments, or sexual difficulties. The severity of Parkinson's symptoms tends to worsen over time.
In the United States, at least 500,000 people are believed to suffer from Parkinson's disease, and about 50,000 new cases are reported annually. These figures are expected to increase as the average age of the population increases. The disorder appears to be slightly more common in men than women. The average age of onset is about 60. Both prevalence and incidence increase with advancing age; the rates are very low in people under 40 and rise among people in their 70s and 80s. Parkinson's disease is found all over the world. The rates vary from country to country, but it is not clear whether this reflects true ethnic and/or geographic differences or discrepancies in data collection.
Treatment of Parkinson's Disease varies from patient to patient but there are several drugs that seem to help with the symptoms of the disease. The most widely used one, Levadopa (L-dopa), is converted into dopamine by the body, thereby increasing the amount of dopamine in the brain and decreasing the symptoms of the disorder. While there currently is no cure for Parkinson's disease, progress in the field is being made. One such promising treatment is deep brain stimulation. In this procedure, an electrode is placed in the patient's brain and then stimulated by a battery pack. The electrical impulses seem to decrease or stop the physical symptoms of the disorder in most patients.
A. Who Gets Parkinson's Disease?
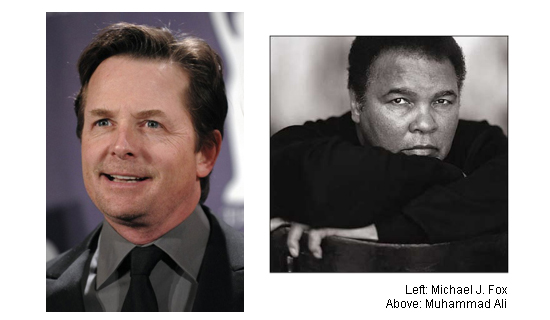
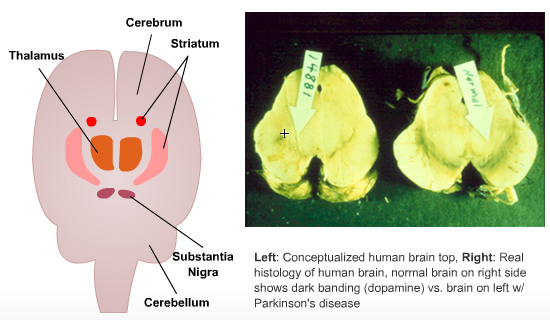
C. What Causes Parkinson's Disease?
- The cause of most cases of Parkinson's Disease is unknown
- Genes and chemicals have been implicated in some cases
D. Can Parkinson's Disease Be Cured?
- No, but it can be treated with drugs and surgery
- Drugs that increase brain dopamine (levadopa) or mimic dopamine (agonists) are most commonly used
- The neurosurgical technique of Deep Brain Stimulation (DBS) is also being used more commonly
2. Symptoms of Parkinson's Disease
Parkinson's Disease is usually diagnosed after the appearance of one or more of its four most common motor symptoms: resting tremor, bradykinesia, rigidity, and postural instability. Parkinson's patients will exhibit any of
these symptoms in a wide range of severity. There are many more symptoms associated with Parkinson's Disease. These symptoms fall into three main categories: primary motor symptoms, secondary motor symptoms, and nonmotor symptoms.
Primary Motor Symptoms
- Resting Tremor - A "resting tremor" is called such because it appears as a slight tremor in a body part that is not at work and disappears when that body part begins an action. About 70% of all Parkinson's patients experience a resting tremor in the beginning stages of the disease.
- Bradykinesia - Bradykinesia refers to the slowing of a person's movements. Parkinson's patients can experience both slow movements and incomplete movements along with difficulty initiating movements or sudden halts to movements in progress. Bradykinesia in Parkinson's patients is often characterized by slow, shuffling steps and a reduction in facial expressions.
- Rigidity - Rigidity (increased muscle tone) leads to stiffness and inflexibility in the muscles. Muscles normally relax when they are at rest and, in the case of rigidity, the muscles never relax, leading to decreased range of motion as well as pain and cramping in some situations. The combination of bradykinesia and rigidity in facial muscles can lead to a "mask-like' look, due to a reduction in facial expressions.
- Postural Instability - Parkinson's patients often experience instability while tanding as well as reduced balance and coordination. This, in combination with bradykinesia, greatly increases the risk of falling and causes difficulty with making turns or sudden movements. These individuals may also experience "freezing", where they can not initiate walking and feel stuck.
Secondary Motor Symptoms
Secondary motor symptoms are called such because, while they are commonly found in cases of Parkinson's Disease, they are found in differing combinations and degrees of severity.
- Dystonia - Involuntary contractions of the muscles, leading to twisting and repetitive movements
- Fatigue
- Impaired Fine Motor Dexterity and Motor Coordination
- Impaired Gross Motor Coordination
- Poverty of Movement - Decreased arm swing
- Akathisia - Feelings of inner restlessness, resulting in the inability to stay still
- Speech Problems - Softness of voice or slurred speech caused by lack of muscle control
- Loss of Facial Expression, or 'Masking'
- Micrographia - Small, cramped handwriting
- Difficulty Swallowing
- Sexual Dysfunction
- Drooling
- Drooped Posture w/ Tendency to Lean Forward
Nonmotor Symptoms
In addition to the many motor symptoms commonly associated with Parkinson's Disease, there are many symptoms exhibited by Parkinson's patients that have nothing to do with movement, hence the term "nonmotor". Again, like the
motor symptoms, these nonmotor symptoms will be exhibited in different combination and in differing degrees of severity by different patients.
- Pain
- Dementia or Confusion
- Sleep Disturbances
- Constipation
- Skin Problems
- Depression
- Fear or Anxiety
- Memory Difficulties and Slowed Thinking
- Urinary Problems
- Fatigue and Aching
- Loss of Energy
- Compulsive Behavior
- Cramping
3. Causes of Parkinson's Disease
It was in the 1960s when the first chemical brain differences were detected in Parkinson's patients. It was discovered that patients showing symptoms of Parkinson's disease had low levels of dopamine in the striatum due to the
loss of dopamine producing neurons in the substantia nigra. The dopaminergic neurons in the substantia nigra have axons that project through the medial forebrain bundle and end in the striatum, where they release dopamine. This
pathway is known as the nigrostriatal tract. The release of dopamine in the striatum then stimulates a series of transmissions, beginning with the thalamus and ending with the motor cortex.
Further research determined that the Parkinson's patients had usually lost over 80% of their dopamine-producing neurons in the substantia nigra before they began showing visible symptoms. What was not known is why these dopamine
neurons begin to die or how the brain can maintain such a great loss of dopamine-producing neurons before showing visible symptoms.
There are several theories as to what causes the initial loss of dopamine-producing neurons in the substantia nigra and it is thought that the cause may vary between patients. It is thought that the disease may at times be the
result of genetics, chemicals, environmental toxins or any combination of the three. Individuals who develop symptoms before the age of 50 are thought to have a stronger genetic tendency than those who develop symptoms later in
life.
4. How Dopamine Neurotransmission Works
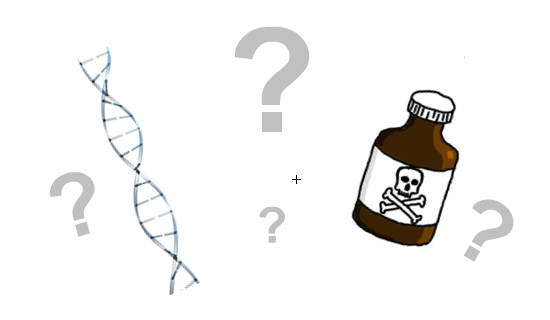
- No, but it can be treated with drugs and surgery
- Drugs that increase brain dopamine (levadopa) or mimic dopamine (agonists) are most commonly used
- The neurosurgical technique of Deep Brain Stimulation (DBS) is also being used more commonly
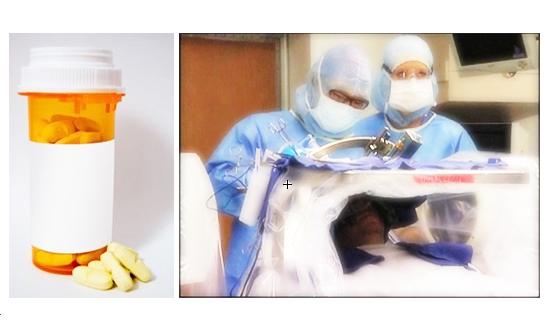
2. Symptoms of Parkinson's Disease
Parkinson's Disease is usually diagnosed after the appearance of one or more of its four most common motor symptoms: resting tremor, bradykinesia, rigidity, and postural instability. Parkinson's patients will exhibit any of
these symptoms in a wide range of severity. There are many more symptoms associated with Parkinson's Disease. These symptoms fall into three main categories: primary motor symptoms, secondary motor symptoms, and nonmotor symptoms.
Primary Motor Symptoms
- Resting Tremor - A "resting tremor" is called such because it appears as a slight tremor in a body part that is not at work and disappears when that body part begins an action. About 70% of all Parkinson's patients experience a resting tremor in the beginning stages of the disease.
- Bradykinesia - Bradykinesia refers to the slowing of a person's movements. Parkinson's patients can experience both slow movements and incomplete movements along with difficulty initiating movements or sudden halts to movements in progress. Bradykinesia in Parkinson's patients is often characterized by slow, shuffling steps and a reduction in facial expressions.
- Rigidity - Rigidity (increased muscle tone) leads to stiffness and inflexibility in the muscles. Muscles normally relax when they are at rest and, in the case of rigidity, the muscles never relax, leading to decreased range of motion as well as pain and cramping in some situations. The combination of bradykinesia and rigidity in facial muscles can lead to a "mask-like' look, due to a reduction in facial expressions.
- Postural Instability - Parkinson's patients often experience instability while tanding as well as reduced balance and coordination. This, in combination with bradykinesia, greatly increases the risk of falling and causes difficulty with making turns or sudden movements. These individuals may also experience "freezing", where they can not initiate walking and feel stuck.
Secondary Motor Symptoms
Secondary motor symptoms are called such because, while they are commonly found in cases of Parkinson's Disease, they are found in differing combinations and degrees of severity.
- Dystonia - Involuntary contractions of the muscles, leading to twisting and repetitive movements
- Fatigue
- Impaired Fine Motor Dexterity and Motor Coordination
- Impaired Gross Motor Coordination
- Poverty of Movement - Decreased arm swing
- Akathisia - Feelings of inner restlessness, resulting in the inability to stay still
- Speech Problems - Softness of voice or slurred speech caused by lack of muscle control
- Loss of Facial Expression, or 'Masking'
- Micrographia - Small, cramped handwriting
- Difficulty Swallowing
- Sexual Dysfunction
- Drooling
- Drooped Posture w/ Tendency to Lean Forward
Nonmotor Symptoms
In addition to the many motor symptoms commonly associated with Parkinson's Disease, there are many symptoms exhibited by Parkinson's patients that have nothing to do with movement, hence the term "nonmotor". Again, like the
motor symptoms, these nonmotor symptoms will be exhibited in different combination and in differing degrees of severity by different patients.
- Pain
- Dementia or Confusion
- Sleep Disturbances
- Constipation
- Skin Problems
- Depression
- Fear or Anxiety
- Memory Difficulties and Slowed Thinking
- Urinary Problems
- Fatigue and Aching
- Loss of Energy
- Compulsive Behavior
- Cramping
3. Causes of Parkinson's Disease
It was in the 1960s when the first chemical brain differences were detected in Parkinson's patients. It was discovered that patients showing symptoms of Parkinson's disease had low levels of dopamine in the striatum due to the
loss of dopamine producing neurons in the substantia nigra. The dopaminergic neurons in the substantia nigra have axons that project through the medial forebrain bundle and end in the striatum, where they release dopamine. This
pathway is known as the nigrostriatal tract. The release of dopamine in the striatum then stimulates a series of transmissions, beginning with the thalamus and ending with the motor cortex.
Further research determined that the Parkinson's patients had usually lost over 80% of their dopamine-producing neurons in the substantia nigra before they began showing visible symptoms. What was not known is why these dopamine
neurons begin to die or how the brain can maintain such a great loss of dopamine-producing neurons before showing visible symptoms.
There are several theories as to what causes the initial loss of dopamine-producing neurons in the substantia nigra and it is thought that the cause may vary between patients. It is thought that the disease may at times be the
result of genetics, chemicals, environmental toxins or any combination of the three. Individuals who develop symptoms before the age of 50 are thought to have a stronger genetic tendency than those who develop symptoms later in
life.
4. How Dopamine Neurotransmission Works
- Pain
- Dementia or Confusion
- Sleep Disturbances
- Constipation
- Skin Problems
- Depression
- Fear or Anxiety
- Memory Difficulties and Slowed Thinking
- Urinary Problems
- Fatigue and Aching
- Loss of Energy
- Compulsive Behavior
- Cramping
3. Causes of Parkinson's Disease
It was in the 1960s when the first chemical brain differences were detected in Parkinson's patients. It was discovered that patients showing symptoms of Parkinson's disease had low levels of dopamine in the striatum due to the
loss of dopamine producing neurons in the substantia nigra. The dopaminergic neurons in the substantia nigra have axons that project through the medial forebrain bundle and end in the striatum, where they release dopamine. This
pathway is known as the nigrostriatal tract. The release of dopamine in the striatum then stimulates a series of transmissions, beginning with the thalamus and ending with the motor cortex.
Further research determined that the Parkinson's patients had usually lost over 80% of their dopamine-producing neurons in the substantia nigra before they began showing visible symptoms. What was not known is why these dopamine
neurons begin to die or how the brain can maintain such a great loss of dopamine-producing neurons before showing visible symptoms.
There are several theories as to what causes the initial loss of dopamine-producing neurons in the substantia nigra and it is thought that the cause may vary between patients. It is thought that the disease may at times be the
result of genetics, chemicals, environmental toxins or any combination of the three. Individuals who develop symptoms before the age of 50 are thought to have a stronger genetic tendency than those who develop symptoms later in
life.
4. How Dopamine Neurotransmission Works
The standard "textbook" account of neurotransmission (and the most common type of neurotransmission) has the communication between neurons happening exclusively at the synapse. (See animation explaining classic synapse-based chemical tranmission.) The dopamine neurons responsible for motor function in Parkinson's patients do not fit this standard model. Work through the animation below to see how dopamine transmission works in the dopamine neurons that are the focus of this study.
5. Dopamine Tone & the Central Question of this Lab
Dopamine tone is the constant level of extracellular dopamine in the brain. Dopamine tone (or "dopaminergic tone") is the nearly constant, low concentration of DA molecules around target neurons. This concentration is also referred to as dopamine tonic level.
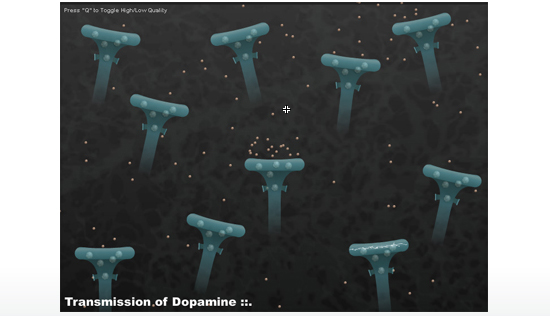
In Parkinson's disease, the loss of dopamine terminals in the striatum leads to a decrease in dopamine tone, resulting in the physical symptoms seen in Parkinson's patients. What is startling, though, is that it appears
that Parkinson's patients can lose up to 80% of these dopamine neurons before seeing a drop in striatal dopamine tone. That means that a Parkinson's patient will have less than 20% of his or her dopamine terminals
remaining in the striatum before symptoms begin to appear. That is very puzzling.
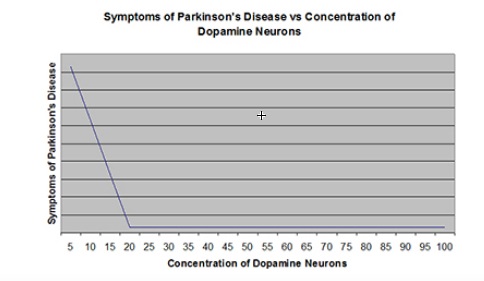
If 80% of the neurons are dead and thus no longer producing dopamine, there must be some mechanism to explain why tonic dopamine remains at healthy levels. Some feature of this process which keeps motor neurons functioning properly must be compensating for the loss of dopamine production by the dopamine terminals that have died. What is that mechanism?
We have come to the central question of this study and the focus of this virtual lab.
What is the mechanism that enables dopamine tone to remain steady (and healthy) despite the loss of up to 80% of the dopamine producing neurons?
Dr. Michael Zigmond, Professor of Neurology at the University of Pittsburgh, was one of early dopamine researchers and one of the first to offer a theory to explain how dopamine tone is maintained even when 80% of dopamine terminals (cells) have died from Parkinson's. His theory is the "increased release theory". This is the most widely held theory today -- but it is not the only theory
6. The Increased Release Theory (the Standard theory)
According to this theory, when dopamine terminals are lost to Parkinson's disease, the remaining dopamine terminals release additional dopamine molecules,
which maintain dopamine tone. At a loss of over 80% of the dopamine terminals, the remaining neurons simply can't produce enough dopamine molecules and the dopamine molecules can no longer diffuse across the now great distance
between active neurons. This is the explanation offered for the motor symptoms exhibited by Parkinson's disease patients.
This animation is designed to help you understand what is thought to be happening at the cellular/molecular level in the "increased release theory" (sometimes called the "compensatory release theory"). This animation
is a conceptual representation of dopamine (DA) cells in the striatum and how they will function when DA cells begin to die off -- assuming, of course, that this theory is true.
As you watch the animation, you'll see that with each action potential, dopamine molecules are released. After being released, many dopamine molecules will be taken up by a dopamine neuron. After being taken up by a dopamine neuron, it
will eventually be repackaged in a vesicle and released again.
As dopmaine neurons are lost, you will notice that the action potentials do not increase in frequency but more dopamine molecules are released with each action potential. This is what Zigmond's "increased release theory" predicts will happen.
7. Experiments to Test Increased Release Theory
A. Zigmond's Hypothesis
Zigmond's "increased release theory" is based on the hypothesis that dopamine release increases to maintain dopamine tone during the preclinical phase of Parkinson's disease, which is the time when DA (dopamine) neurons have begun dying off but before obvious motor deficits begin to appear. The prediction is that as dopamine neurons die off, the remaining healthy neurons will increase the quantity of dopamine molecules that are released at each site.
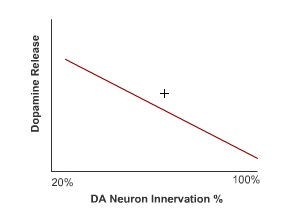
B. Experimental Design
The experiment will measure dopamine tone, dopamine release and dopamine uptake in a rat model of Parkinson's disease. The model of Parkinson's disease is created by "lesioning" which is injecting neurotoxin in the substantia nigra of a rat. This neurotoxin destroys dopamine neurons by generating hydrogen peroxide. The neurotoxin enters the dopamine neuron via a dopamine uptake site. Once in the cell, it reacts with vitamin C to make hydrogen peroxide, which then kills the cell body.
Once the dopamine neurons have been destroyed, you will then need to stimulate the remaining neurons and record their dopamine release and uptake. This is achieved by implanting electrodes in strategic areas of the rat brain.
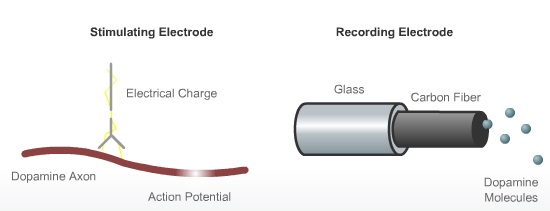
C. What the Experimental Data Shows
The graph below reflects the data gathered during several experimental trials, including the data you gathered if you completed the Virtual Parkinson's Lab. The results show that when there is a high concentration of dopaminergic (DA) neurons, the amount of dopamine released is also high (see upper right hand side of the graph). As the percentage of dopaminergic neurons decreases, so does the percentage of dopamine being released. According to this data, the remaining dopaminergic neurons do not increase dopamine output when dopaminergic neurons begin to die. The data gathered from these studies disconfirm the "increased release theory". Assuming these conclusions are reliable, then there must be some other explanation for why dopaminergic tone remains at healthy levels even when a majority of DA neurons have died. Enter: Paul Garris' "passive stabilization theory".
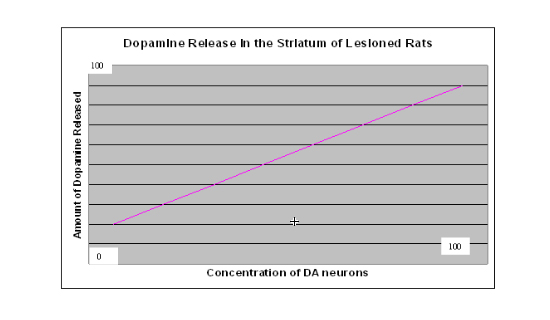
8. The Passive Stabilization Theory
Dr. Garris agrees with Dr. Zigmond that there must be some kind of "compensatory mechanism" that can explain why dopamine tone is not reduced even when 80% of the dopamine neurons have died. Garris, however, does not believe that the "increased release theory" explains this because with new methods for measuring dopamine output from dopamine neurons he was able to measure dopamine release at the neuron sites (see section 7. above). The data shows that the remaining neurons do NOT release more dopamine molecules to compensate for the loss of other neurons. There must be another explanation.
Dr. Garris offers an alternative theory to explain the phenomenon. He proposes the "passive stabilization theory" as the actual compensatory mechanism that preserves dopamine tone even with the death of a large percentage of dopamine neurons. This is explained in the animation below. As you watch the animation, you'll see that with each action potential, a consistent number of dopamine molecules are released. After being released, a dopamine molecule will either be taken by a dopaminergic neuron or glial cell.
If taken up by a dopaminergic neuron, it will be repackaged in a vesicle and eventually released again.
As dopmainergic neurons are lost, you will notice that the action potentials do not increase in frequency nor are more dopamine molecules
released with each action potential.
9. The Bucket Analogy
Paul Garris offers an analogy to show how the "passive stabilization theory" can explain why dopamine tone does not fall below "healthy" levels until the loss of dopamine neurons exceeds 80%. The bucket in the Bucket "Theory" is an analogy as to how passive stabilization works in the striatum. Because it is a rough analogy, it is designed to examine passive stabilization conceptually and not quantitatively. This is why there are few numbers associated with the animation.
The image below shows which features of the "bucket" animation are being compared to which parts of human brain's dopamine system.
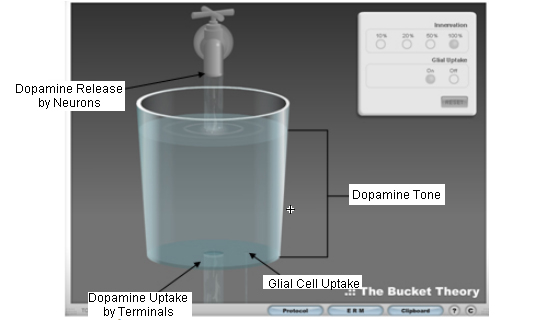
As can be seen above, each part of the bucket analogy represents a main component in the passive stabilization theory. The faucet represents the release of dopamine by dopaminergic neurons, the central drain represents the uptake of dopamine by dopaminergic neurons, the small drain on the right represents dopamine uptake by glial cells and the liquid in the bucket represents dopaminergic tone.
As the level of innervation (= the % of healthy DA neurons) decreases, you'll notice that the faucet and central drain also decrease in diameter, representing the loss of dopamine neurons to both release and take up dopamine. The small drain to the right, however, does not change in size.
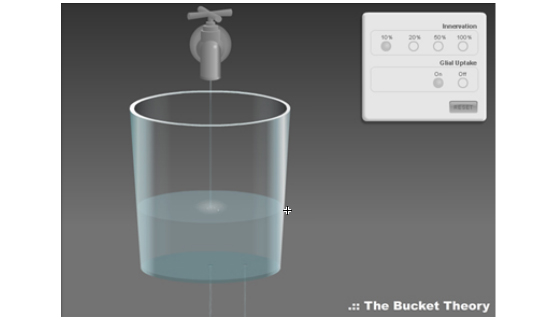
As can be seen in the figure above, by the time innervation reaches 10%, the rate of dopamine uptake by the glial cells, combined with the dopamine uptake by the remaining dopamine neurons, begins to overcome the amount of dopamine released by the dopamine neurons. The result is a decrease in dopmaniergic tone in the striaum -- as the level of water in the bucket begins to go down.
Use the animation below, working through each of the % innervation values to see how dopaminergic tone is affected as the percentage of healthy dopamine neurons increases and decreases.
10. Computer Model of Passive Stabilization
Paul Garris created a computer model based on the passivie stabilization theory. This computational model shows the relationship between (1) dopamine neuron innervation (i.e., what percentage of neurons are still alive, (2) the frequency of action potentials (= neuron firings), and (3) dopamine tone. The computer model may seem confusing at first glance but once you understand what the different elements represent, it can help to demonstrate how dopamine neuronal activity performs if the passive stabilization theory is correct.
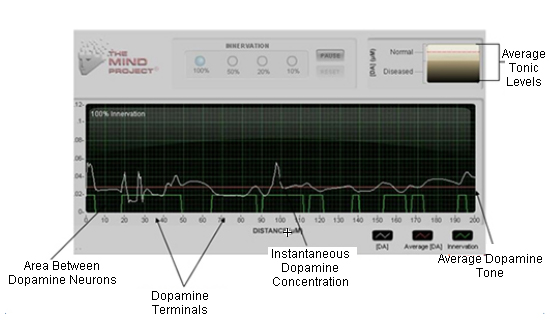
Starting at the bottom of the diagram, you will see green bars with spaces in between. The green bars represent dopamine neurons and the spaces in between them are the areas between the dopamine neurons. Moving further up, you will see a white, wavy line. This white line represents the dopamine concentration that results from release and uptake by individual neurons. Notice that there is dopamine present even in the areas that don't have a dopamine synapse. Finally, the red line represents the average concentration of dopamine in the extrasynaptic (outside of the synapses) space. This concentration is also known as dopamine tone.
Unlike the other animations you've looked at, this computer model is not intended to only conceptually represent the passive stabilization theory but is based directly on data generated in experiments in Dr. Garris' lab.
11. Three-Way Animation: Neurons, Computer Model & Bucket
Now we will put all of the elements together so that you can see how the activity of dopamine neurons in Parkinson's patients fits the "bucket analogy" and matches the computer model. Together these help to explain and support the plausibility of the "passive stabilization theory" as to the neuronal mechanisms at work that explain why Parkinson's patients do not start showing obvious forms of motor disfunction until over 80% of the dopamine neurons have died. Click on each of the "innervation" level buttons (10%, 20%, 50% or 100%) to see how the passive stabilization theory explains the empirical data acquired from the studies described in this lab. (The "innervation" level means the percentage of living dopamine neurons in the patient.)